Introduction:
Nucleic acids are huge macromolecules that are necessary for all cells and viruses to function. The storing and expression of genetic information is a major function of nucleic acids.
It is a naturally occurring chemical molecule that can be broken down to release phosphoric acid, sugars, and an organic base combination (purines and pyrimidines). Nucleic acids are the cell’s major information-carrying molecules, and they control protein synthesis, determining the hereditary traits of all living things.
Friedrich Miescher first separated “nuclein” from the nuclei of pus cells in 1869. Because nuclein was discovered to have acidic qualities, it was given the name nucleic acid.
Class of Nucleic acid:
Deoxyribonucleic acid (DNA) and ribonucleic acid (RNA) are the two major nucleic acids. DNA is found in the nucleus, with trace amounts in mitochondria and chloroplasts. Whereas, RNA is found throughout the cell.
DNA, or deoxyribonucleic acid, encodes the information that cells require to produce proteins.
Ribonucleic acid (RNA), a related type of nucleic acid, exists in a variety of molecular configurations and is involved in a variety of cellular functions, including protein production.
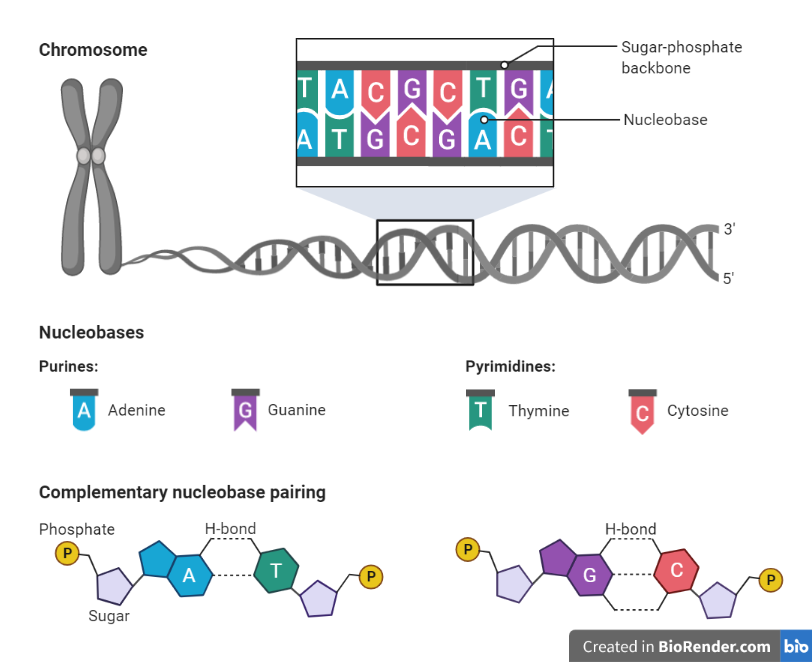
Fig: Different bases of DNA and its structure
The function of nucleic acid:
In the cells of all living things, each type of nucleic acid performs a different function.
DNA
In the body, DNA is responsible for storing and coding genetic information. The structure of DNA helps individuals to inherit genetic information from their parents.
Because the nucleotides adenine, thymine, guanine, and cytosine in DNA may only couple in a specific order (adenine with thymine, and guanine with cytosine), when a cell repeats the strand of DNA, the nucleotides can be copied in a specific order. As a result, precise DNA copies can be created and transmitted down from generation to generation. The instructions for all of the proteins that an organism will produce are contained in DNA.
DNA encodes all of an organism’s instructions for growth, survival, and reproduction. DNA sequences must be transformed into messages that can be utilized to build proteins, which are the complex molecules that do the majority of the work in our bodies, in order to carry out these activities.
RNA
The basic function of RNA is to translate information into proteins. RNA transmits genetic information, which is translated into diverse proteins required for biological functions by ribosomes. The three main forms of RNA involved in protein synthesis are mRNA, rRNA, and tRNA. RNA is also the genetic material that viruses use to reproduce. RNA editing, gene control, and RNA interference are some of the other tasks. Small regulatory RNAs, including as short nuclear RNA, microRNA, and small interfering RNA, are involved in these processes.
- During protein production, it acts as an adaptor molecule.
- Facilitate the translation of DNA into proteins.
- Between the DNA and the ribosomes, it acts as a messenger.
- In all living cells, they are the carriers of genetic information.
- Aid ribosomes to select the appropriate amino acid for the synthesis of new proteins in the body.
Nucleotides: building blocks of nucleic acids:
The structure of nucleic acid:
DNA and RNA are polymers (often very long polymers in the case of DNA) made up of monomers called nucleotides. A polynucleotide (poly- Significance “many”) is formed when these monomers unite to form a chain.
A nucleotide is made up of three molecules that includes a phosphate group, a 5-carbon sugar, and a nitrogen base.
Phosphate group
The phosphate group is made up of a phosphorus atom with four negatively charged oxygen atoms attached to it.
5-carbon sugar
The 5-carbon sugar (known as a pentose) includes ribose and deoxyribose, which are present in nucleic acid. Both ribose and deoxyribose have five carbon atoms and one oxygen atom. Attached to the carbon atoms are hydrogen atoms and hydroxyl groups.
In ribose sugar, there are hydroxyl groups attached to the second and third carbon atoms. In deoxyribose sugar, there is a hydroxyl group attached to the third carbon atom, but only a hydrogen atom is attached to the second carbon atom.
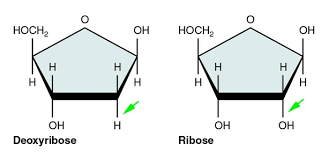
Fig: Ribose and deoxyribose sugar of nucleic acid
Nitrogen base
The nitrogen molecule acts as a base in nucleic acid that can bind to carbon, hydrogen, and oxygen molecules to create ring structures.
Ring structures come in single rings (pyrimidines) and double rings (purines). Pyrimidines include thymine, cytosine, and uracil. Purines include adenine and guanine. Purines are larger than pyrimidines, and their size differences help to determine their pairings in DNA strands.
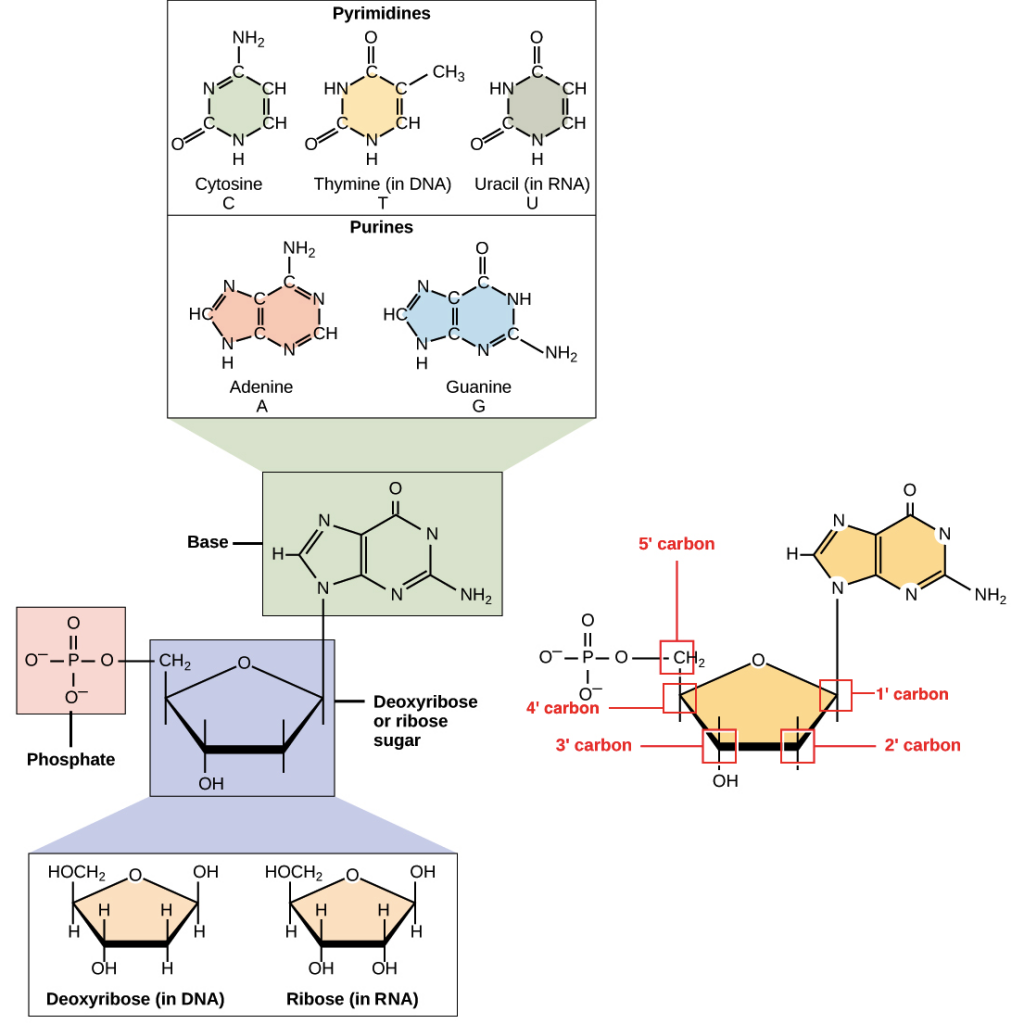
Fig: Nitrogenous bases
Deoxyribonucleic acid (DNA):
Where is DNA found?
DNA is found inside the nucleus in organisms known as eukaryotes. Each DNA molecule must be tightly packaged because the cell is so small and organisms have so many DNA molecules per cell. The packaged form of DNA is named as a chromosome.
During replication, DNA unwinds so that it may be replicated. At other points in the cell cycle, DNA unwinds so that its instructions can be used to make proteins and other biological processes. During cell division, on the other hand, DNA is in its compact chromosomal form, allowing it to be transported to nearby cells.
Nuclear DNA is the term given to DNA present in the nucleus of a cell. The genome refers to an organism’s entire set of nuclear DNA.
Humans and other complicated organisms have a little quantity of DNA in cell structures called mitochondria, in addition to the DNA in the nucleus. Mitochondria produce the energy required for a cell to function properly.
Organisms receive half of their nuclear DNA from their male parent and half from their female parent during sexual reproduction. Organisms, on the other hand, get all of their mitochondrial DNA from their female parent. This happens because during reproduction, only egg cells, not sperm cells, retain their mitochondria.
Who discovered DNA?
In the late 1800s, Swiss biologist Frederich Miescher discovered DNA for the first time. However, it took nearly a century for researchers to figure out the structure of the DNA molecule and understand its significance in life. Scientists fought for years about which molecule conveyed life’s biological blueprints. The majority of scientists believed that DNA was far too simple a molecule to fulfill such a crucial function. Instead, they claimed that because of their increased complexity and variety of forms, proteins were more likely to perform this critical function. Because of the contribution of James Watson, Francis Crick, Maurice Wilkins, and Rosalind Franklin, the significance of DNA was recognized in 1953. The double helix structure of DNA, which allows it to convey biological information from one generation to the next, was discovered by researching X-ray diffraction patterns and developing models.
Properties of DNA:
DNA is a two-stranded helix with hydrogen bonds linking the two strands together. Chargaff’s rule holds that A bases are always paired with Ts and Cs are always paired with Gs.
The majority of DNA double helices are right-handed; that is, if you hold your right hand out with your thumb pointed up and your fingers curled around your thumb, your thumb represents the helix’s axis and your fingers represent the sugar-phosphate backbone. Left-handed DNA is found only in one form of DNA, Z-DNA.
The 5′ end of one strand is connected with the 3′ end of its corresponding strand in the DNA double helix, which is anti-parallel (and vice versa).
Phosphate groups connect the 3′ end of one sugar to the 5′ end of the next sugar, and this is how nucleotides are linked.
The outer edges of the nitrogen-containing bases are exposed and available for possible hydrogen bonding, in addition to the DNA base pairs. Other molecules, particularly proteins that serve critical functions in DNA replication and expression, can easily access the DNA because of these hydrogen bonds.
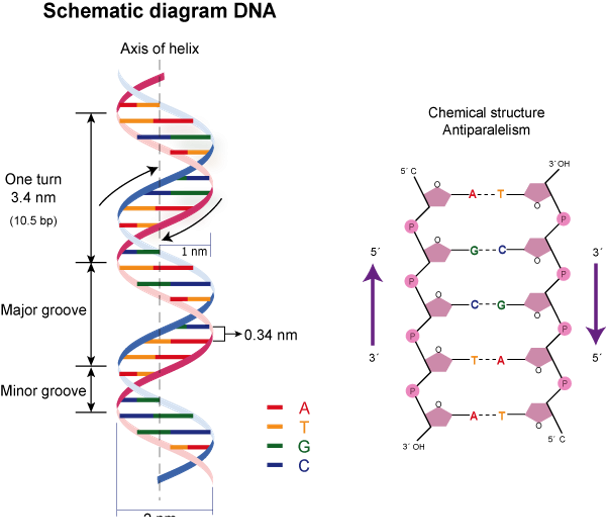
Fig: Structure of DNA
Types of DNA:
There are three different forms of DNA:
A-DNA
It is a double helix that is identical to B-DNA in that it is right-handed. When DNA is dehydrated, it takes on the shape of an A, which protects it from harsh circumstances like dehydration. Protein binding also removes the solvent from DNA, causing it to take the shape of an A.
B-DNA
B-DNA is a right-handed helix that is the most prevalent DNA shape. Under normal physiological settings, the majority of DNA conforms to the B type.
C-DNA
When DNA is exposed to low humidity and certain ions such as Li+ and Mg2+, it takes on this shape. This is an unstable form that doesn’t exist in nature. The nucleotide conformations in B and C-DNA are similar, but the ratios are different.
D-DNA
It is an uncommon variation that is missing the Guanine (G) base unit. When compared to A-DNA, this type of DNA occurs when the humidity is lower.
E-DNA
It is a type of organismal DNA that is found in the environment and is extended or eccentric in nature. E-DNA is derived from cellular material such as skin, mucus, excreted feces, hair, gametes, and corpses that are shed into the environment by different creatures. Depending on environmental factors such as acidity, heat, and radiation, DNA can live anywhere from 7 to 21 days.
Z-DNA
This is left-handed DNA with a zig-zag pattern on the double helix. Andres Wang and Alexander Rich were the ones who found it. It is thought to play a role in gene regulation since it is present ahead of the start location of a gene.
Physical and Chemical Properties of DNA:
ABSORPTION
- UV radiation with a wavelength of 260 nanometers absorbs the bases in DNA.
- A spectrophotometer can measure the amount of absorption.
- One approach for calculating the concentration of DNA in a solution is to utilize this method.
- More ultraviolet light is absorbed the less ordered the bases are.
- 1.60 units at 260 nm are absorbed by free bases.
- At a wavelength of 260 nm, single-stranded DNA absorbs 1.37 units.
- At 260 nm, double-stranded DNA absorbs one unit.
DENSITY
- CsCl will produce a density gradient when ultracentrifuged, with the denser solution at the bottom.
- DNA and other macromolecules will clump together in a region of CsCl with the same density as them.
- As a result, denser DNA will migrate downwards, while less dense DNA will migrate upwards, resulting in bands.
- The density of GC base pairs is higher than the density of AT base pairs. As a result, DNA with a higher proportion of GC base pairs will form bands lower down than DNA with a higher proportion of AT base pairs.
- When chromosomal DNA is sliced into roughly equal fragments and centrifuged with CsCldensity, two bands emerge.
- The majority of the genome’s DNA is contained in one band.
- The second band (the satellite) has a highly repetitive sequence and contains around 5% of the genome’s DNA.
DENATURATION
- When a double-stranded DNA molecule is split into two single-stranded molecules, it is referred to as denatured DNA. The increase in ultraviolet light absorption can be examined to see if this is the case.
- The frequency with which hydrogen bonds between molecules break rises as thermal energy increases.
- The two molecules split into single-stranded molecules as the temperature rises. A DNA molecule’s Tm (melting temperature) is the temperature at which half of its molecules denature.
- A DNA molecule’s G+C content can be estimated using the Tm. Because G-C base pairs are bound together by three hydrogen bonds (compared to two for A-Ts), separating molecules with high GC concentrations requires more energy (higher temperatures).
HYDROPHOBICITY OF SOLVENT
- The Tm of DNA molecules is reduced by hydrophobic substances.
- DNA bases will dissolve into the solvent when exposed to hydrophobic substances.
- As a result, the bases are not constrained to being stacked on top of one another, making it easier to break the hydrogen bonds that hold DNA molecules together.
- Hydrophilic substances have a tendency to raise DNA’s Tm.
- These will keep DNA bases stacked on top of each other in the most favorable position for hydrogen bonding between DNA strands.
pH
- Phosphodiester linkages between nucleotides and the N-glycosidic bond between the sugar and purine bases are disrupted at pHs lower than one.
- When the pH is approximately 4, the N-glycosidic linkages between the sugar and purines are selectively weakened.
- Because the purines have been removed from the DNA, it is referred to as apurinic acid.
- The polarity of groups involved in hydrogen bonding is changed by alkali bases.
- All hydrogen bonds break at pH 11.3, and DNA is completely denatured.
- To a pH of about 13, DNA is resistant to hydrolysis.
- It is hydrolyzed unless it is apurinic.
- Around pH 11, RNA is hydrolyzed into ribonucleotides.
IONIC STRENGTH
- The phosphates in the sugar-phosphate backbones of DNA are negatively charged, thus they oppose each other. DNA in distilled water will denature spontaneously into single-stranded DNA.
- The charges of the phosphate groups are neutralized by dissociating salts into ions.
- The DNA double helix will be stabilized, resulting in a greater Tm.
- G+C content is usually about 0.5 in higher organisms (0.49 – 0.51 for primates)
- Some bacteria have lower organisms that vary from 0.27 to 0.76.
- The thermal melting temperature of a DNA molecule can be used to calculate its G+C content (Tm).
SOLUBILITY
- In comparison to DNA, RNA is more soluble in water.
- Deoxyribose has a 2′-H group while ribose has a 2′-OH group.
- RNA is less stable than DNA because hydrooxyl groups are polar and dissolve better in water. C-H is a non-polar link and so hydrophobic.
- The hydroxyl group on ribose’s 2′ carbon is more reactive than deoxyribose’s hydrogen.
SIZE
- The sugar-phosphate backbone contains negatively charged phosphates.
- When DNA is exposed to an electrical field, it tends to gravitate toward the positive electrode (the cathode)
- Smaller fragments of DNA travel quicker through a gel than larger amounts of DNA.
- Larger fragments have a difficult time squeezing through the gel matrix and are thus delayed, whereas smaller pieces migrate more quickly.
VELOCITY SEDIMENTATION
- The speed of sedimentation is determined by two factors: density and form.
- The denser the DNA, the faster it settles during centrifugation.
- Linear molecules will sediment faster than globular (more compact) ones.
ELECTRON MICROSCOPY
- Electron microscopy can be used to determine the size of DNA molecules.
- The DNA is displayed on a grid of known size in order to estimate the size of the DNA molecule.
- Concentration of DNA
- Absorption At 260 nm, DNA absorbs UV light.
- The higher the absorption, the more DNA there is.
- By comparing absorption to known DNA D values, DNA concentrations can be approximated.
RENATURATION
- The process of annealing the two complementary DNA strands together is known as DNA renaturation. It will eventually happen as a result of cooling. Renaturation occurs when hydrogen bonds between complementary base pairs are reformed, bringing the two DNA strands together to form double-stranded DNA.
- Renaturation can be achieved by gradually reducing the temperature or by introducing ions to the solution.
- The rate of renaturation is influenced by the amount of DNA present.
Ribonucleic acid (RNA):
RNA structure
RNA typically is a single-stranded biopolymer. However, the presence of self-complementary sequences in the RNA strand leads to intrachain base-pairing and folding of the ribonucleotide chain into complex structural forms consisting of bulges and helices.
An important structural component of RNA that distinguishes it from DNA is the presence of a hydroxyl group at the 2′ position of the ribose sugar.
Types of RNA:
RNA molecules play a variety of roles in the cell. The major types of RNA are:
Ribosomal RNA (rRNA)
The most abundant kind of RNA in the cell is ribosomal RNA (rRNA).
Transfer RNA (tRNA)
It is the second most abundant type of RNA.
Messenger RNA (mRNA)
This is a type of RNA that transmits information to the ribosome about the amino acid sequence of a protein. The only type of RNA that is translated is messenger RNA.
Heterogeneous nuclear RNA (hnRNA or pre-mRNA)
This form of RNA is found solely in eukaryotic cells’ nuclei. It is made up of mRNA precursors that are produced during posttranscriptional processing.
Small nuclear RNA (snRNA)
It is also only found in the nucleus of eukaryotes. One of its major functions is to participate in splicing (removal of introns) mRNA.
Micro-RNA (miRNA)
It is a short, non-coding, ~ 22 nt long, at least some of which control the expression or repression of other genes during development. Their major role is in the posttranscriptional regulation of protein expression.
Ribozymes
This is the form of RNA molecules with enzymatic activity. They are found in both prokaryotes and eukaryotes.
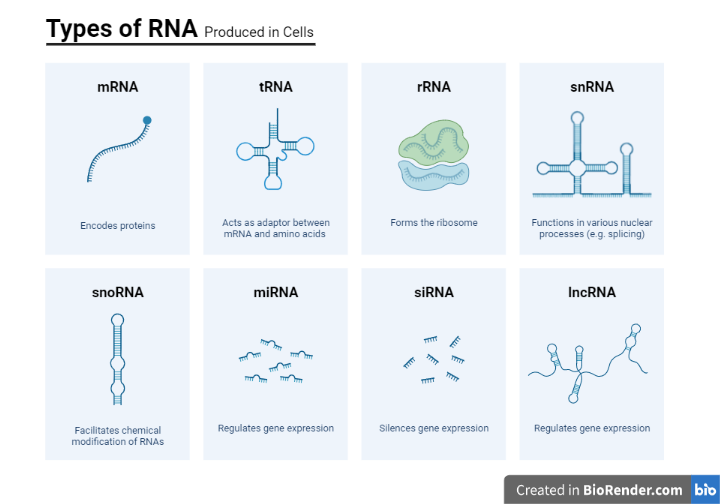
Fig: Different types of RNA
RNA in Comparison with DNA:
RNA has a molecular structure that is extremely similar to DNA, yet it differs in three major ways:
- RNA, unlike double-stranded DNA, is usually a single-stranded molecule (ssRNA) that consists of significantly shorter chains of nucleotides in many of its biological tasks. However, as in tRNA, double-stranded RNA (dsRNA) can develop, and a single RNA molecule can create intra strand double helixes through complementary base pairing.
- RNA has ribose instead of deoxyribose in its sugar-phosphate “backbone.”
- In contrast to deoxyribose, ribose has a hydroxyl group connected to the pentose ring in the 2′ position. The hydroxyl groups in the ribose backbone lower the activation energy of hydrolysis, making RNA more chemically labile than DNA.
- In DNA, thymine is the complimentary nucleotide to adenine, however in RNA, it is uracil, which is an unmethylated version of thymine.
Application in diagnosis and therapy:
Nucleic acid testing, also known as nucleic acid amplification testing (NAT or NAAT), is a procedure that includes amplifying and detecting genetic material (nucleic acids, DNA or RNA) for diagnosis or therapy recommendations. Despite the fact that every living being’s genetic material is made up of DNA or RNA, genome sequences differ. Because of this genetic variety, NAT is an excellent approach for detecting infectious infections, cancer, genetic abnormalities, and mitochondrial disorders, as well as for assisting personalized and precision therapy based on pharmacogenomics information.
Depending on the condition being investigated, NAT can be conducted on a variety of clinical specimens. NAT specimens include blood, plasma, serum, cerebrospinal fluid, sterile bodily fluids, sputum, urine, stool, and tissues. Manual or automated procedures are used to extract nucleic acids from the cell and other biological components. The extracted nucleic acids can subsequently be amplified and detected, or sequences read, using a variety of techniques, including polymerase chain reaction (PCR), real-time PCR, microarrays, and sequencing (Sanger and next-generation), among others. The uses of NAT have changed dramatically because to advancements in technology for amplification, detection, and sequencing.
Summary:
Nucleic acid is a component of all living things and serves as the foundation for DNA and RNA. It’s found in every cell, as well as several viruses. Nucleic acids have a wide range of tasks, including cell formation, genetic information storage and processing, protein synthesis, and energy cell generation.
Despite the fact that their roles differ, DNA and RNA have remarkably similar structures, with just a few fundamental variations in their molecular make-up distinguishing them.
References:
- Nelson, D.L., Lehninger, A.L. and Cox, M.M., 2008. Lehninger principles of biochemistry. Macmillan.
- Satyanarayana, U., 2021. Biochemistry, 6e-E-book. Elsevier Health Sciences.
- Chargaff, E. ed., 2012. The nucleic acids. Elsevier.
- Pray, L. (2008) Discovery of DNA structure and function: Watson and Crick. Nature Education 1(1):100
- Dahm, R. Discovering DNA: Friedrich Miescher and the early years of nucleic acid research. Human Genetics 122, 565–581 (2008).
- David Hames and Nigel Hooper (2005). Biochemistry. Third ed. Taylor & Francis Group: New York.
- Alberts, B. et al. Molecular biology of the cell. (2002).
- Adams, R.L. ed., 2012. The biochemistry of the nucleic acids. Springer Science & Business Media.
- Cheatham III, T.E. and Kollman, P.A., 2000. Molecular dynamics simulation of nucleic acids. Annual review of physical chemistry, 51(1), pp.435-471.