Introduction:
The tricarboxylic acid (TCA) cycle, also known as the Krebs or citric acid cycle, is the most important central metabolic pathway that is the prominent source of energy to the body.
The Krebs cycle is responsible for producing 65–70% of the ATP. This cycle is responsible for the production of the majority of the reduced coenzymes used for the generation of ATP in the electron transfer chain.
Acetyl CoA is largely oxidized throughout the citric acid cycle to produce CO2 and H2O.Approximately two-thirds of the body’s total oxygen intake is used up during this cycle. Since tricarboxylic acids (citrate, cis-aconitate, and isocitrate) is the first stable intermediate synthesised in the cycle, it is also termed as TCA cycle. The TCA cycle is a component of the broader glucose metabolism, in which glucose is oxidized to produce pyruvate, which is subsequently oxidized and enters the TCA cycle as acetyl-CoA.
Cellular respiration:
A metabolic process called cellular respiration converts glucose to ATP. Glycolysis, pyruvate oxidation, the citric acid or Krebs cycle, and oxidative phosphorylation are the phases of cellular respiration.
It occurs in three major stages
In the first stage, the organic fuel molecules such as glucose, fatty acids, and certain amino acids are oxidized to produce two-carbon fragments in the form of the acetyl group of acetyl-coenzyme A. (acetyl-CoA).
The acetyl groups are transported into the citric acid cycle in the second stage, where they are enzymatically converted to CO2 and the energy released is stored in the reduced electron carriers NADH and FADH2.
The third stage of respiration involves the oxidation of these reduced coenzymes, which releases protons (H+) and electrons. Through a network of electron-carrying molecules known as the respiratory chain, the electrons are delivered to O2, the final electron acceptor. By a process known as oxidative phosphorylation, the significant quantity of energy generated during electron transport is preserved in the form of ATP.
History:
This cycle was first identified in 1937 by German chemist Hans Adolf Krebs, who also made other important biochemical discoveries. In 1953, Krebs was awarded the Nobel Prize in Physiology or Medicine for his work on the study of intermediate metabolism in the oxidative breakdown of carbohydrates. After receiving a rejection from Nature, Krebs and his co-author William Arthur Johnson published their research in Enzymologia under the title “The role of citric acid in intermediate metabolism in animal tissues.”
Location:
The enzymes of TCA cycle are located in mitochondrial matrix, in close proximity to the
electron transport chain.
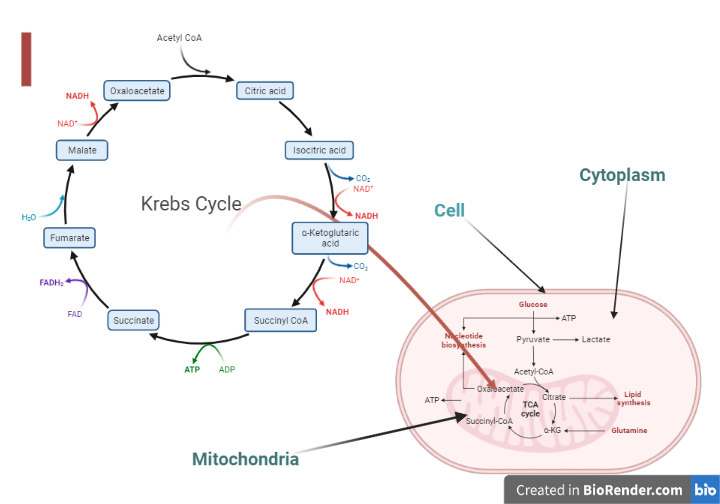
Fig: Location of TCA cycle
Amphibolic nature:
The citric acid cycle provides a diverse range intermediate for the synthesis of several chemicals required by the organism. Being both catabolic and anabolic in nature, the Krebs cycle is described as amphibolic.
The citric acid cycle, which is used in both catabolic and anabolic processes in aerobic organisms, is an amphibolic pathway. In addition to helping in the oxidative degradation of carbohydrates, fatty acids, and amino acids, the cycle also acts as a precursor for numerous metabolic processes.
This series of reactions not only provides the majority of the energy required by complex organisms, but the molecules generated in these reactions also serve as the building blocks for a variety of critical processes, such as the synthesis of fatty acids, steroids, cholesterol, amino acids for the construction of proteins, and the purines and pyrimidines required for DNA synthesis.
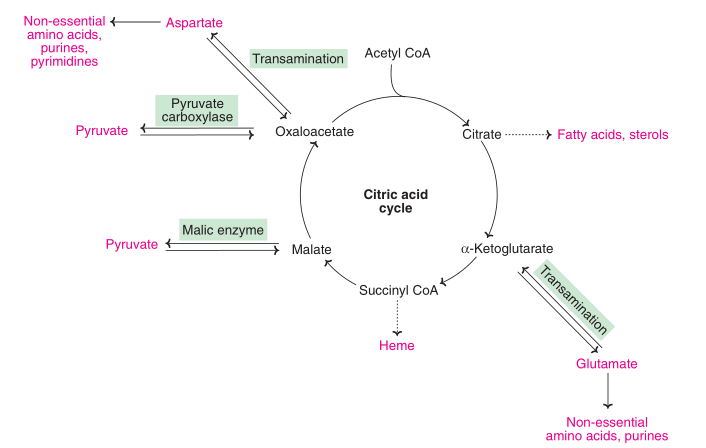
Fig: Major synthetic pathways of the intermediates of citric acid cycle
Steps:
Formation of citrate
The TCA cycle begins with an enzymatic aldol addition reaction of acetyl CoA to oxaloacetate, forming citrate.
Citrate is isomerized to isocitrate
Isocitrate is produced when the citrate is isomerized by a dehydration-hydration process.
Formation of α-ketoglutarate
Further enzymatic oxidation and decarboxylation gives 2-ketoglutarate.
Conversion of α-ketoglutarate to succinyl CoA
α-ketoglutarate is converted into succinyl-CoA through an additional enzymatic decarboxylation and oxidation.
Formation of succinate
The phosphorylation of guanosine diphosphate (GDP) to guanosine triphosphate occurs simultaneously with the hydrolysis of the metabolite to succinate (GTP).
Conversion of succinate to fumarate
Fumarate is produced by succinate dehydrogenase, an enzyme that is dependent on the flavin adenine dinucleotide (FAD).
Formation of malate
After stereospecific hydration, fumarate catalysed by fumarase is transformed to L-malate.
Conversion of malate to oxaloacetate
The last step of NAD-coupled oxidation of L-malate to oxaloacetate is catalysed by malate dehydrogenase and closes the cycle.

Fig: Steps of TCA cycle and their inhibitors
Energetics of citric acid cycle:
Four reducing equivalents—three in the form of NADH and one in the form of FADH2 are generated during the oxidation of acetyl CoA via the citric acid cycle. In contrast to FADH2, which produces 2 ATP, 3 NADH is oxidized by the electron transport chain and followed by oxidative phosphorylation to produce 9 ATP. Additionally, there is phosphorylation at the substrate level. Thus, one acetyl CoA yields a total of twelve ATP.
Significances:
- The last process for oxidizing glucose, lipids, and amino acids is known as the Krebs cycle or citric acid cycle.
- As an alternative energy source, many animals depend on nutrients besides glucose.
- Deaminated amino acids are transformed to pyruvate and other Krebs cycle intermediates as a metabolic by-product of proteins. They enter the cycle and are metabolized, for example, aspartate is deaminated to form oxaloacetate, alanine to pyruvate, and glutamate to α-ketoglutarate. Fatty acids undergo ?-oxidation to form acetyl CoA, which enters the Krebs cycle
- It is the primary mechanism through which cells produce ATP. Following complete oxidation of nutrients, a significant amount of energy is generated.
- It is crucial for the processes of gluconeogenesis, lipogenesis, and amino acid interconversion.
- Amino acids, nucleotides, cytochromes, chlorophylls, and other chemicals are all synthesized using a variety of intermediary substances.
Regulations:
Allosteric regulation controls the TCA cycle. By using feedback inhibition, the metabolites control the cycle. Controlling the rate of the citric acid cycle absolutely depends on the cellular requirements for ATP. Either enzyme activity or ADP concentrations are responsible for the control. The citric acid cycle is regulated by three enzymes: citrate synthase, isocitrate dehydrogenase, and alpha-ketoglutarate dehydrogenase.
- Acetyl CoA, succinyl CoA, NADH, ATP, and NADH all are responsible to inhibit citrate synthase.
- Isocitrate dehydrogenase is inhibited by ATP and NADH and activated by ADP.
- NADH and succinyl CoA both inhibit alpha-Ketoglutarate dehydrogenase.
- The citric acid cycle requires the presence of ADP in order to proceed. This is because oxidation of NADH and FADH2 through the electron transport chain interruption without enough ADP, which is associated with phosphorylation of ADP to ATP. The concentration of NADH and FADH2 inhibits the enzymes and reduces the availability of NAD+ and FAD, which are required for the TCA cycle to continue.
Diseases:
- When the Krebs cycle is disrupted, normal energy synthesis is inhibited, which results in a variety of metabolic abnormalities and symptoms. This disruption can be brought on by a lack of energy substrates, hereditary or acquired illness conditions, or physical stress.
- Tricarboxylic acid cycle (TCA cycle) illnesses are a subset of extremely rare human conditions that impact basic mitochondrial metabolism.
- The fumarate hydratase enzyme’s dysfunction is the primary reason of the fumarase deficit. Developmental delays, severe mental retardation, linguistic difficulties, seizures, and facial dysmorphism are all signs of the illness.
- When succinate dehydrogenase is lacking, mitochondrial complex II, which connects the TCA cycle to the electron transport chain, is impacted. The phenotypic can include Leigh syndrome, leukodystrophy, cardiomyopathy, and a decline in mental and physical abilities.
- Alpha-ketoglutarate dehydrogenase deficiency is a fatal early childhood disease marked by encephalopathy and hyperlactatemia. It is incredibly rare.
References:
- Morava, E., Carrozzo, R. (2014). Disorders of the Krebs Cycle. In: Blau, N., Duran, M., Gibson, K., Dionisi Vici, C. (eds) Physician’s Guide to the Diagnosis, Treatment, and Follow-Up of Inherited Metabolic Diseases. Springer, Berlin, Heidelberg.
- Alabduladhem, T.O. and Bordoni, B., 2021. Physiology, Krebs Cycle. In StatPearls [Internet]. StatPearls Publishing.
- Satyanarayana, U., 2021. Biochemistry, 6e-E-book. Elsevier Health Sciences.
- David, L., Nelson, D.L., Cox, M.M., Stiedemann, L., McGlynn Jr, M.E. and Fay, M.R., 2000. Lehninger principles of biochemistry.
- Fernie, A.R., Carrari, F. and Sweetlove, L.J., 2004. Respiratory metabolism: glycolysis, the TCA cycle and mitochondrial electron transport. Current opinion in plant biology, 7(3), pp.254-261.