Introduction:
- A biophysical process known as electroporation occurs when externally administered pulsed electric fields are used to improve the permeability of cell membranes. There are numerous uses for this increase in membrane permeability in biotechnology, medicine, and the food business.
- An effective, non-viral delivery method, electroporation, also known as electropermeabilization that enables molecules such as medicines, proteins, genetic material (DNA and RNA), and other compounds to enter cells.
- In electroporation, an electric current is applied to a living surface, such as the skin or a cell membrane that open pores or channels through which is used to introduce foreign DNA or other molecules into cells. It works by temporarily creating small openings in the cell membrane, through which DNA or other molecules or drugs can be introduced.
- Transfection is a more general term that refers to the process of introducing any type of foreign genetic material into cells, which can be achieved through various methods, including electroporation, chemical transfection and physical transfection (viral infection, lipofection)
- This is accomplished by applying a short electrical pulse to the cells, which causes the membrane to become permeable. Once the electrical pulse is removed, the openings in the membrane reseal, trapping the introduced molecules inside the cell. Transfectants are cells that have been transformed with foreign DNA using a technique such as electroporation. The term can also refer to the DNA itself that has been introduced into the cells.
Principle:
The basis of electroporation is the capacity of electrical field to briefly permeate the cell membrane, allowing for the reception of foreign molecules like DNA or RNA.
A conductive fluid containing host cells and a selected molecules is encircled by an electrical circuit. The cell suspension is discharged by an electrical pulse that is discharged at an optimal voltage and for a few milliseconds to few microseconds. By generating an electrical gradient across the cell membrane when an electric field is given to a cell, the electrical gradient has the potential to destabilize the membrane and lead to the formation of pores. Once the electric field is removed, these pores quickly close since they are transient.

Fig: Principle of electroporation
The pores created during electroporation are small, usually on the order of a few nanometers, but large enough to allow the passage of small molecules like DNA or RNA through the cell membrane. The opening and closing of these pores is a dynamic process, and the efficiency of electroporation can be influenced by factors such as the strength and duration of the electric field, the type of cells being transfected, and the properties of the foreign molecules being introduced.
Requirements:
A suitable cell types
Electroporation is most commonly used with prokaryotic cells, such as bacteria, and eukaryotic cells, such as animal and plant cells. The type of cell and its membrane structure will affect the conditions necessary for successful electroporation.
Adequate cell viability
Electroporation is a physical process that can damage cells, so the cells to be electroporated should be healthy and viable. To ensure adequate cell viability during electroporation, it is important to optimize the electroporation conditions and use healthy and viable cells
There are several factors that can affect cell viability during electroporation:
- High-voltage pulses
- Cell concentration
- Conductive medium
- Pre-electroporation treatment: Some treatments, such as freezing and thawing, can damage cells and make them more susceptible to damage during electroporation.
- Post-electroporation treatment: The cells need to be incubated under the appropriate conditions to allow them to recover after the electroporation process.
Electroporator
Electroporators are devices that use electricity to permeate cell membranes. These tools are frequently employed in transfection and transformation processes. The components of electroporators are:
- Electrodes: The electrodes are used to deliver the electrical pulses to the cells. They are typically placed on opposite sides of the cells and the electrical pulses are applied across them.
- Pulse control unit: This component is used to control the parameters of the electrical pulses, such as amplitude, duration, and pulse shape.
- Electrode holder: This component holds the electrodes in place and ensures that they are properly positioned relative to the cells.
- Power supply: This component provides the power necessary to generate the electrical pulses.
Some modern electroporator have other feature such as temperature control, pressure control, data logging and data analysis.
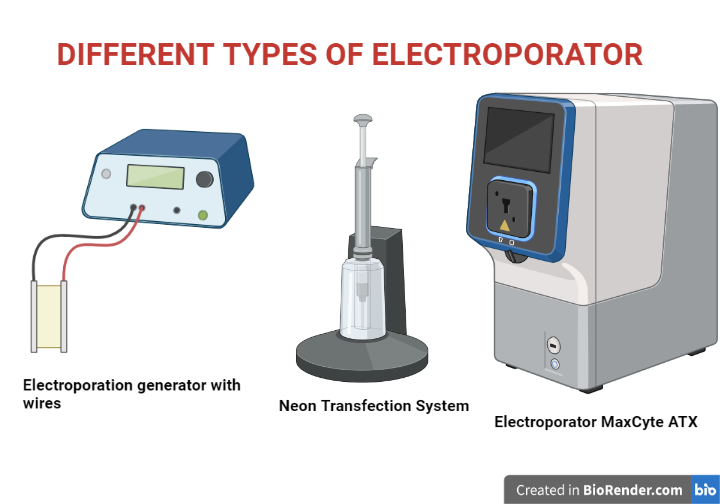
Fig: Different types of electroporators
Selective conductive medium
A selective conductive medium is a solution that is used in electroporation to increase the efficiency of the electroporation process by selectively permeabilizing the target cells while leaving other cells unharmed.
There are various ways to achieve selectivity, one of the way is by using different buffer solutions containing different types of ions such as KCl, NaCl and CaCl2, depending on the type of cells being electroporated. The membrane potentials and the conductivity of the buffer solutions affect the electroporation process, making some cells more permeable to the electrical pulses than others.
Another way is to use some chemical agents that selectively permeabilize certain types of cells. For example, some membrane-active agents, such as nystatin, polyethylene glycol (PEG) or liposomes, can be added to the conductive medium to increase the efficiency of electroporation in certain types of cells.
It is also possible to use of certain antibodies or other molecules that specifically bind to receptors on the surface of target cells, thereby enabling the electrical pulses to selectively permeabilize the target cells while leaving other cells unharmed.
Types:
Classical electroporation: This is the most basic form of electroporation, in which a constant electric field is applied to a cell or tissue sample for a certain period of time. This method is mainly used for in vitro applications, and is typically used to introduce molecules such as DNA or small interfering RNA (siRNA) into cells.
Cell-penetrating electroporation: This method is used to introduce large molecules or nanoparticles into cells, such as proteins or plasmids. This method uses intense electric fields and short pulse duration, which are able to disrupt the cell membrane and allow for the entry of larger molecules.
Gene electro transfer: This method is used for in vivo applications, where it is used to introduce DNA or RNA into cells within a living organism. Gene electro transfer uses lower electric fields and longer pulse duration to minimize tissue damage, and is used to treat a variety of genetic diseases.
Constant field electroporation: This is the most basic form of electroporation, in which a constant electric field is applied to a cell or tissue sample for a certain period of time.
Pulsed field electroporation: This method involves applying a series of brief electric pulses to the sample, rather than a constant field.
Reversal electroporation: This method involves applying an electric field to a cell or tissue sample, and then reversing the field.
AC field electroporation: This method is similar to constant field electroporation, but with an alternating current of electricity instead of a direct current, which have been shown to be more effective in some cases.
In vivo electroporation: This method of electroporation is applied to cells and tissue in a living organism, as opposed to in vitro electroporation which is applied to cells and tissue samples removed from an organism.
Tissue electro transfer: This method is used for in vivo applications to introduce DNA or RNA into specific tissue types such as muscles, skin, or tumor. Also uses lower electric fields and longer pulse duration to minimize tissue damage, with the aim of treating a number of different medical conditions.
Reversal electroporation:
Reversal electroporation is a technique that uses electric fields to temporarily permeabilize cell membranes in the opposite direction of conventional electroporation, in order to remove molecules that are already inside the cells.
During reversal electroporation, a brief, high-voltage electric pulse is applied across the cell membrane in the opposite direction of the pulse used in conventional electroporation. This causes the pores that were formed during conventional electroporation to close and expels molecules that have entered the cell, such as proteins, plasmids or other foreign molecules.
Reversal electroporation is a relatively new technique, but it has potential applications in a variety of fields, including cell-based therapies, where it can be used to remove toxic molecules from cells before transplantation, also it is being researched as a method of drug delivery to remove drugs from cells after they have been used.
Additionally, Reversal Electroporation can be used as a powerful genetic tool for in-vivo gene editing by removing gene editing molecules such as CRISPR ribonucleoproteins after performing their editing task.
The differences between conventional and reversal electroporation are-
- Conventional electroporation and reversal electroporation are both techniques that use electric fields to permeabilize cell membranes, but they differ in the direction of the applied electric field.
- In conventional electroporation, an electric field is applied to cells in order to increase the permeability of their membrane. This allows for the introduction of foreign molecules, such as DNA or drugs, into the cells. The electric field is applied in a way that creates transient openings in the cell membrane, called “pores,” which allow for the uptake of molecules.
- In reversal electroporation, the electric field is applied in the opposite direction, which causes the pores to close. This technique is mainly used to remove molecules that are already inside the cells.
- While electroporation has wide range of uses in laboratory and biomedical field, one of the main applications of reversal electroporation is in cell-based therapies, such as Chimeric antigen receptor (CAR) T and in that way, deliver large molecules like proteins and plasmids. It also used for removing toxic molecules from cells before transplantation.
Steps:
Cell preparation: The cells to be transfected are grown in culture and then harvested and washed to remove any residual growth media.
Cell resuspension: The cells are resuspended in an electroporation buffer at a concentration suitable for the particular type of cells being used.
Mixing with plasmid DNA: The cells are mixed with the plasmid DNA to be transfected, or with other molecules like siRNA or RNA that you want to introduce into the cells.
Loading into electroporation cuvette: The cell-DNA mixture is loaded into an electroporation cuvette, which is a small, thin-walled container designed for electroporation.
Electroporation: The cuvette is placed in an electroporator, a device that delivers the electric pulse. The pulse creates temporary pores in the cell membrane, allowing the foreign DNA to enter the cell. In addition, electrical pulse creates a potential difference across the cell membrane and induces temporary pores in the membrane for nucleic acid entry.
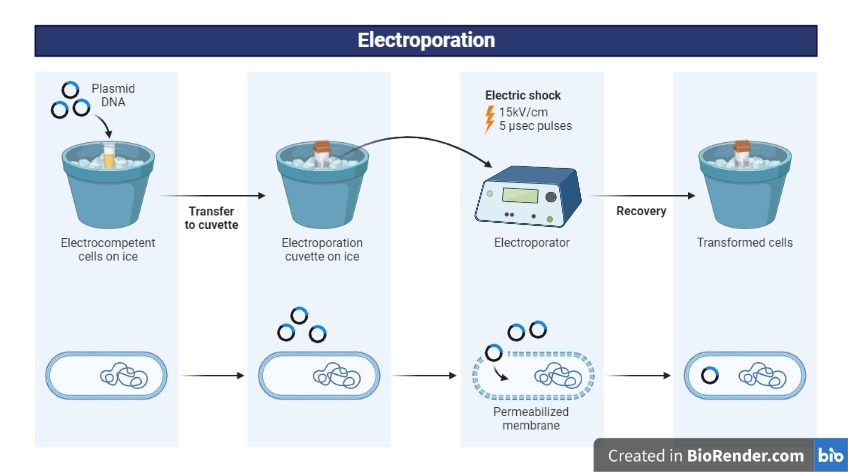
Fig: Bacterial electroporation Transformation
Recovery and selection: The cells are then moved to fresh culture media and allowed to recover. Depending on the type of plasmid being transfected, cells that have taken up the plasmid may be selected using antibiotic resistance.
Harvesting and analysis: After a sufficient time, the cells are harvested and analyzed to determine the success of the electroporation.
Returning cells to growing condition: Return cells to growth conditions and allow them to recover.
Assay cells: Assay cells for gene expression or silencing.
Protocol:
- Prepare a cell suspension in a suitable buffer (e.g., PBS) at the appropriate concentration.
- Mix the cell suspension with the DNA or RNA to be introduced (usually at a ratio of 1 μg of DNA per 106 cells).
- Transfer the mixture of cell and DNA to a sterile electroporation cuvette.
- Apply a short electrical pulse (e.g., 200 V, 25 ms) using an electroporator.
- Immediately transfer the cells to a recovery medium (e.g., DMEM containing 10% FBS) and incubate for a suitable period of time (e.g., 24 hours) to allow for the cells to recover.
- Analyze the cells for expression of the introduced gene or phenotype change.
Applications:
Gene delivery: Electroporation is an efficient method for delivering DNA, RNA, and other genetic materials into cells, making it an important tool for genetic engineering and gene therapy research.
Vaccines: Electroporation has been proposed as a new way to improve the efficiency of DNA and protein vaccines by increasing the number of antigens that reach the cells and by triggering a stronger immune response.
Cancer research: Electroporation has been used to introduce anticancer drugs or genes encoding for cancer-fighting proteins into cancer cells.
Protein delivery: Electroporation can be used to deliver proteins, enzymes, and other biomolecules into cells, which is useful for studying protein function and for applications such as enzyme replacement therapy.
Plant cells: Electroporation has been used to introduce DNA into plant cells in order to genetically engineer crops and study the functions of plant genes.
Bacterial transformation: Electroporation is a widely used method for transforming bacteria with plasmid DNA, which allows for efficient genetic engineering of bacteria.
Cosmetics and Food industry: Electroporation has been used to introduce specific molecules or enzymes to achieve a desired effect in the final product, like the production of cosmetics and food with specific properties.
Bioremediation: Electroporation has been used to introduce DNA encoding for enzymes involved in the degradation of pollutants into bacteria.
Biological samples analysis: Electroporation has been used to lyse cells and make their content available for further analysis, such as PCR and sequencing.
Cancer Tumor Electrochemotherapy: Researchers are looking into how electroporation can improve the efficacy of chemotherapy. The administered electrical pulse would damage the tumor cell’s membrane, similar to how electroporation for DNA transfection does, and enhance the amount of medicine supplied to the spot. According to several research, when this approach is used to treat malignant cells in animal model systems, tumors are reduced more effectively.
Gene therapy: Using electroporation techniques, essential genes can be carried through the skin and into the target tissue, much like medicine delivery. The protein created by this gene may replace a damaged one if it was integrated into body cells, treating a hereditary disease.
Advantages:
High efficiency: Electroporation can result in high transfection efficiencies, meaning a large proportion of cells can take up the introduced material. Without affecting viability, a significant proportion of cells are transfected.
Selective delivery: Electroporation can be used to target specific types of cells, allowing for selective delivery of materials.
Minimal damage to cells: The electrical pulses used in electroporation are brief, which can help to minimize damage to cells.
Versatility—Electroporation can be employed in several cell types
Flexibility—A range of cell numbers can be used: from 104 to 106 cells
Non-toxic: Unlike some other methods for introducing materials into cells, electroporation does not rely on chemical agents that may be toxic to cells.
Versatility: Electroporation can be used to introduce a wide variety of materials into cells, including DNA, RNA, and proteins.
Scalability: Electroporation can be used with cells in culture, or with isolated cells or tissue samples, and can be easily scaled from small scale to large scale.
Disadvantages:
Cell death: Electroporation can cause some degree of cell death, as the electrical pulses can damage cells. This can be especially problematic for cells that are already fragile or in poor health.
Variability: Results can be inconsistent, as the rate of transfection can vary depending on the cell type and the conditions used.
Equipment and Expertise: Electroporation requires specialized equipment and typically needs experienced personnel to be done properly.
Expensive: Specialized equipment, such as electroporator, and reagents can be expensive.
Limited range of cells: Electroporation may not be effective for all types of cells. For example, some types of primary cells and stem cells are difficult to transfect using electroporation.
Storage: After electroporation the cells have to be handled carefully, for example with cooling or other preservation methods.
Effect on the cell’s activity: After electroporation, cells may experience an inhibitory effect on their activity. This may not be useful if the cells are needed to perform specific functions, such as producing proteins. The use of high voltage to conduct electro transfer in internal organs is challenging due to the need for surgical operation and the risk of organ damage.
References:
- Towhidi L, Khodadadi D, Maimari N, Pedrigi RM, Ip H, Kis Z, Kwak BR, Petrova TW, Delorenzi M, Krams R. Comparison between direct and reverse electroporation of cells in situ: a simulation study. Physiol Rep. 2016 Mar;4(6): e12673.
- https://www.thermofisher.com/np/en/home/references/gibco-cell-culture-basics/transfection-basics/methods/electroporation.html
- Tylewicz, U., 2020. How does pulsed electric field work?. In Pulsed electric fields to obtain healthier and sustainable food for tomorrow (pp. 3-21). Academic Press.
- https://www.technologynetworks.com/cell-science/articles/an-introduction-to-electroporation-a-tool-for-transfection-and-competent-cell-generation-363195
- Kotnik, T., Frey, W., Sack, M., Meglič, S.H., Peterka, M. and Miklavčič, D., 2015. Electroporation-based applications in biotechnology. Trends in biotechnology, 33(8), pp.480-488.