Introduction:
- An incredible rate of progress in genetic engineering is producing several benefits. Through the new ability to accurately alter and modify the genomes of living creatures and by advancing and accelerating the study of functional genomics, genome editing technology has transformed the approach of genetic and biological research.
- For some time, the science of genome engineering has seen the emergence of high precision genome editing tools like RNA-guided engineered nucleases (RGENs), Zinc finger nucleases (ZFNs), and Transcription-activator-like effector nucleases (TALENs).
- However, the introduction of CRISPR-Cas9 technology ignited a great deal of excitement around the globe. This new generation of genome editing tools has a range of advantages that set it apart from competing genome editing tools and earning it the title of “breakthrough of the year 2015.”
- The application is primarily used in the study of transcriptional activation/repression, live-cell imaging of DNA localization, and the creation of genetic knock-out mutants in a variety of model systems, including Droshophila melanogaster (common fruit fly), Bombyxmori (silkworm), Caenorhabditis elegans (roundworm), Daniorerio (zebrafish), and Musmusculus (house mouse). A promising tool for biomedical research and the therapy of diverse genetic abnormalities is thought to be the CRISPR-Cas system.
- In the field of biomedical research and the treatment of numerous genetic problems, the CRISPR-Cas technology is seen to be a promising approach.
What Is CRISPR-Cas9?
- It is an effective method for editing genomes, enabling scientists to quickly change DNA sequences and affect gene function. It offers a wide range of potential uses, including the correction of chromosomal anomalies, the treatment and prevention of illness, and the enhancement of crop growth, adaptability and many more. Even so, the technology also presents numerous ethical issues and questions despite its promise.
- CRISPR stands for “clustered regularly interspaced short palindromic repeats,” which are distinctive DNA sequences discovered in some bacteria and other microbes. These sequences constitute an immune system that protects against viruses and other infectious DNA, together with the genes that are situated near to them and are referred to as CRISPR-associated or Cas genes.
- Foreign DNA is recognised, cut off, and removed by the CRISPR system. Five different kinds of CRISPR systems have been categorized by researchers. The CRISPR-Cas9 system, which is connected to the Cas9 protein, is the most researched CRISPR system. Researchers improved CRISPR-Cas9 in 2012 and 2013 to function as a technique that is effective and efficient for editing the genomes of plants, animals, and microbes. Since that moment, the genomes of numerous species, including corn and yeast as well as mice and fruit flies, have been altered using the CRISPR-Cas9 system.
CRISPR-Cas9 as a Adaptive Immune:
Invading bacteriophages are protected against bacteria by the CRISPR-Cas9 system. As spacers within the bacterial genome’s CRISPR locus, Cas1 and Cas2 incorporate a brief section of the viral genome after infection. The CRISPR locus is expressed as pre-CRNA and tracrRNA upon re-infection with the same virus. This pre-crRNA undergoes processing to produce guide RNAs (gRNAs), which bind the ribonucleoprotein Cas9 and corresponding portions of the invading virus genome, causing Cas9 to cleave the genome.

History:
- The innovative CRISPR-Cas9 method was first discovered in 1987, the beginnings of one of biology’s most significant discoveries.
- Dr. Emmanuel Charpentier of the Max Planck Unit for the Science of Pathogens in Berlin and Dr. Jennifer Doudna of the University of California, Berkeley collaboratively made the discovery of CRISPR. In 2012, they released a pioneering publication in the journal Science that demonstrated how the CRISPR-Cas9 bacterial immune system might be used to alter genes.
- In 2020, Doudna and Charpentier were the first all-female team to win the Nobel prize in chemistry for their invention, years after it had been widely used in laboratories throughout the world.
- Feng Zhang, who pioneered the use of CRISPR in eukaryotic cells and found novel Cas variations, at the Broad Institute of MIT and Harvard in Cambridge, Massachusetts, is another important contributor. In addition, it was originally used in human cells by George Church of Harvard Medical School in Boston, Massachusetts.
Mechanism:
The primary purpose of the CRISPR-Cas system is to initiate a double-stranded break in the target DNA, allowing for the insertion of a new gene or gene of interest. Generally, two essential components make up the CRISPR-Cas9 system, which modifies the targeted DNA which included:
An enzyme (Cas9)
This acts as a pair of ‘molecular scissors’ that can cut the two strands of DNA at a specific location in the genome so that to allow for the addition or deletion of DNA fragments.
A piece of RNA (Guide RNA, gRNA)
It is a short (20 bases or so) pre-designed RNA sequence fragment that is incorporated within a larger RNA scaffold. The pre-designed sequence “guides” Cas9 to the appropriate region of the genome while the scaffold component binds to DNA which ensures that the Cas9 enzyme performs a cut at the appropriate location in the DNA.
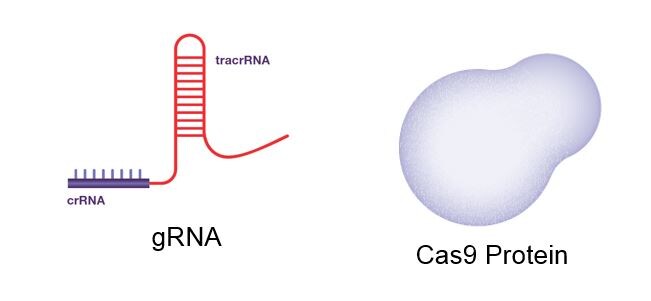
Fig: The CRISPR gRNA and Cas9 Protein
The basic mechanism of CRISPR involves several steps:
Target identification
The first step in CRISPR-based gene editing is to identify the specific location in the genome that you want to target for modification. This is typically done using short RNA molecules called guide RNAs (gRNAs), which are designed to bind to a specific sequence in the genome.
Cas9 binding
The gRNA and Cas9 enzyme are introduced into the cell together. The gRNA recognizes and binds to the target sequence in the genome, and brings the Cas9 enzyme to the same location.
DNA cleavage
Once the Cas9 enzyme is bound to the target sequence, it uses its endonuclease activity to cut both strands of the DNA double helix at the target site. This creates a double-stranded break (DSB) in the genome.
Repair
The cell’s natural DNA repair mechanisms are then activated to repair the DSB. There are two main pathways for repair: non-homologous end joining (NHEJ) and homology-directed repair (HDR). NHEJ is a less accurate repair pathway that often results in small insertions or deletions (indels) at the cut site. HDR is a more accurate repair pathway that requires a template DNA molecule to guide the repair process.
Gene modification
Depending on the specific CRISPR protocol being used, the repair process can result in the modification of the target gene. For example, CRISPR interference (CRISPRi) uses a modified version of Cas9 to inhibit gene expression without making any permanent changes to the genome. CRISPR knockout uses the Cas9 enzyme to permanently delete a specific gene from the genome. CRISPR base editing uses a modified version of Cas9 to make specific, targeted changes to the DNA base pair at a specific location in the genome.

Source: Genome Research Limited.
Fig: The working mechanism of CRISPR-Cas9 editing tool
A potent tool for genome engineering is CRISPR/Cas9. The protospacer is a particular sequence that the Cas9 complex with sgRNA can identify. This is only possible if this sequence is followed by a Protospacer Adjacent Motif (PAM). A dsDNA break results from Cas9 binding. Consequently, non-homologous end joining or homology directed repair will proceed that result in mutations or modifications to genes, respectively.
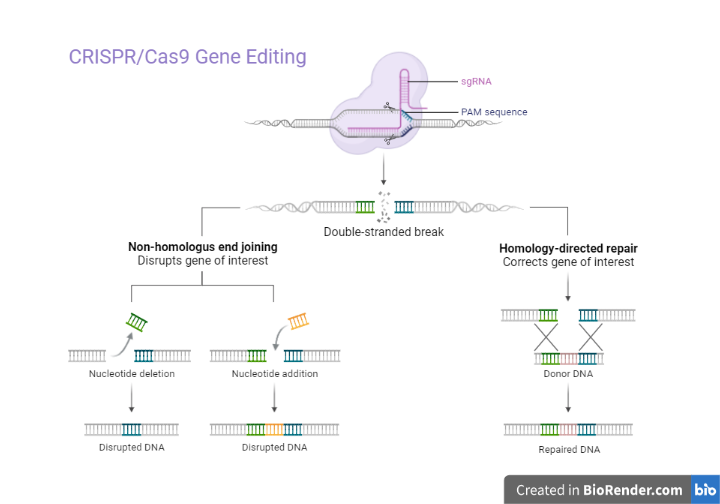
Fig: CRISPR/Cas9 Gene Editing
Applications:
There are many different applications for the CRISPR-Cas9 system. It has been used in medical research on pathogen detection, viral infections, cancer, and hereditary illnesses.
In addition, it has the potential to drastically revolutionize agriculture by increasing the nutritional value and yield of plants and making them more resistant to biotic and abiotic stress that will aid to meet the demands of an expanding global population.
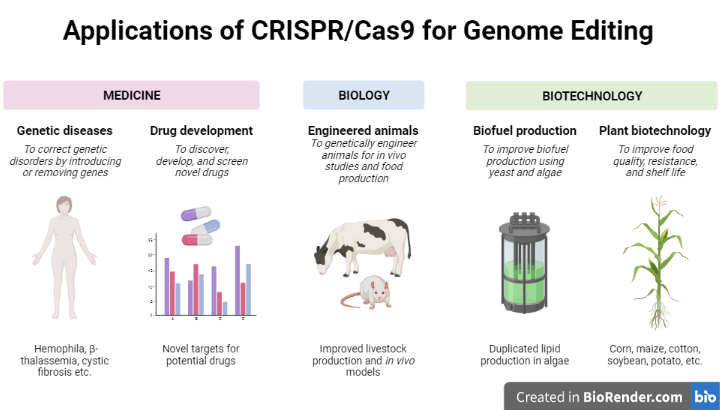
Fig: Applications of CRISPR Technology
Future:
Numerous researchers are constantly coming up with new ideas and methodologies to use CRISPR-Cas9 gene editing technology to address issues in the real world, such as epigenome editing, novel cell and gene therapies, infectious disease research, and the preservation of endangered species. There are also an increasing number of biotech start-up companies specializing in CRISPR-Cas9 gene editing technology.
- Gene therapies: CRISPR could be used to edit the genome of cells in order to correct genetic defects that cause diseases such as sickle cell anemia and muscular dystrophy.
- Cancer therapies: CRISPR could be used to target and delete specific genes that contribute to the development and progression of cancer, or to boost the immune system’s ability to fight cancer.
- Agricultural applications: CRISPR could be used to develop crops with improved yields, resistance to pests and diseases, and tolerance to drought and other environmental stresses.
- Environmental applications: CRISPR could be used to modify the genomes of species such as mosquitoes, which are vectors for diseases such as malaria, in order to reduce the transmission of these diseases.
- Industrial applications: CRISPR could be used to engineer microorganisms for use in the production of drugs, chemicals, and other materials.
References:
- Mohanty, S., Dash, A. and Pradhan, C.K., 2019. CRISPR-Cas9 Technology: A magical tool for DNA editing. Cellular Physiology, 1(1), pp.1-11.
- Ratner HK, Sampson TR, Weiss DS. Overview of CRISPR–Cas9 biology. J Cold Spring Harbor Protocols 2016;2016: pdb. top088849.
- Hsu PD, Lander ES, Zhang F. Development and Applications of CRISPR-Cas9 for Genome Engineering. Cell 2014; 157:1262–78.
- Torres-Ruiz R, Rodriguez-Perales S. CRISPR-Cas9 technology: applications and human disease modelling. Brief Funct Genomics. 2017 Jan;16(1):4-12. doi: 10.1093/bfgp/elw025. Epub 2016 Jun 26. PMID: 27345434.
- Addgene. (2017) Chapter 3: Using CRISPR in Your Experiments. CRISPR 101:
- Djekoun, A., 2021. Therapeutic and diagnostic relevance of Crispr technology. Biomedicine & Pharmacotherapy, 138, p.111487.
- Kim S, Ji S, Koh HR. CRISPR as a Diagnostic Tool. Biomolecules. 2021 Aug 6;11(8):1162. doi: 10.3390/biom11081162. PMID: 34439828; PMCID: PMC8391464.