Introduction:
A biosensor is an analytical device, which employs a biological material to specifically interact an analyte; this interaction procedures some detectable physical change that is measured and converted into an electrical signal by a transducer. Finally, the electrical signal is amplified, interpreted, and displayed as analyte concentration in the solution/preparation.
An analyte is a compound whose concentration is determined, in this case, by the biosensor.
- The biological materials used are usually an enzyme, but nucleic acids, antibodies, lectins, whole cell, entire organs or tissue slices are also used.
- The nature of interaction between analyte and the biological materials used in the biosensor may be of the two types-
- The analyte may be converted into a new chemical molecule (by enzyme; such biosensors are called catalytic biosensors.
- The analyte may simply bind to the biological material present on the biosensor (e.g., to antibodies, nucleic acids; these biosensors are known as affinity biosensors.
Features of Biosensor:
- Highly specific.
- The reaction used should be as independent of factors like stirring, pH, temperatures, etc., as is mergeable.
- The device should be tiny and biocompatible, in case it is to be used for analytes within the body.
- The device should be cheap, small and easy to use.
- It should be durable, i.e., should be capable of repeated use.
- The assay should be rapid, accurate, and repeatable.
- It should be robust, stable, and sterilization.
Components of Biosensor:
A biosensor has the following two types of components.
- Biological (Enzyme antibody, etc.)
- Physical (Transducer, amplifier, etc.)
The biological component of biosensor performs the following functions:
- It specifically recognizes the analyte
- Interacts with it in such manner, which produces some physical change detectable by the transducer. These biological features provide the biosensor its specialized sensitivity and ability to detect and analyze the analyte.
The biological component is suitability immobilized on to the transducer in one of the following ways.
- Enzymes are usually immobilized by glutaraldehyde onto a porous sheet like lens tissue paper or nylon net fabric, and the enzyme-membrane so produced is affixed to the transducer. Generally, a correct immobilization of the enzymes enhances their stability, which may be rather dramatic in some cases. As a result, many enzyme-immobile systems can be utilized over 10,000 times over the course of many months.
- The biological component may be held next to the transducer by a membrane that allows the analyte into this compartment but does not permit the biological component to move out of it. The membrane, if carefully made or selected, may increase the specifically and reduce side reactions.
- Â The biological component, e.g., enzyme, may be adsorbed onto a porous carbon electrode. In such cases, the enzyme is likely to leach out of the electrode.
- The transducer surface may be treated with 3-aminopropyltriethoxysilane. The biological component may now be covalently linked to this cross-linked siloxane via the reactive amnio groups that remain free. This method yields nonreproducible results and often causes a large reduction in the activity of the biological component.
The biological component interacts precisely with the analyte, causing a physical change close to the transducer surface. This physically change may be
- Calorimetric biosensor- Heat released or absorbed by the reaction.
- Potentiometric biosensor- An electrical potential is produced as a result of a shift in electron dispersion.
- Amperometric biosensor- Movement of electrons due to a redox reaction.
- Optical biosensor- Light emitted or absorbed during the reaction
- Acoustic wave biosensor- The change in mass of the biological component caused by the reaction.
The transducer detects and measures the change, converting it into an electrical signal. This signal is always extremely small and is amplified by an amplifier before being delivered into the microprocessor. The signal is subsequently analyzed, interpreted, and displayed in appropriate units. Thus, biosensors convert a chemical information flow into an electrical information flow through the following stages:
The analyte diffuses from the fluid to the surface of the biosensor.
The analyte responds specifically and efficiently with the biological component of the biosensor.
The reaction changes the physiochemical properties of the transducer surface.
This causes a change in the optical or electrical properties of the transducer surface.
The change in optical/electronic characteristics is measured, transformed into an electrical signal, and then amplified, processed, and displayed.
Types of Biosensors:
Calorimetric Biosensor
These biosensors are also called thermometric biosensors or thermal biosensors. Many enzymes catalyzed reactions produce heat (exothermic). Calorimetric biosensors assess temperature changes in the solution containing the analyte as a result of enzyme action and interpret these in terms of analyte concentration. The analyte solution is passes through a small packed bed column containing the immobilized enzyme; the temperature of the solution is determined just before entry of the solution into the column and just as it is leaving the column using separate thermistors. The difference in the temperatures is usually as fraction of one degree Celsius, but it is possible to detect differences as low as 0.00001 degree Celsius.
This is most applicable type of biosensor and cane be used for turbid and strongly colored solutions.
Potentiometric Biosensors
These biosensors use ion selective electrodes to transform biological reactions into electrical signals. The electrodes employed are most commonly pH meter glass electrodes, glass pH electrodes coated with a gas selective membrane or solid sate electrodes. Many reactions generate or use up H+, which is detected and measured by the biosensor; in such cases, very weakly buffered solutions are used. Gas sensing electrodes detect and quantify the amount of gas generated. An example of such an electrode is based on the urease (pH 6.0), which catalyzes the following reactions using as substate.
 Amperometric Biosensors
When a potential is applied between two electrodes, the electrodes generate a current, the magnitude of which is proportional to the substrate concentration. The simplest amperometric biosensor uses the Clark oxygen electrode, which determines the reduction of 02 present in the sample (analyte) solution. These are the first-generation biosensors. These biosensors are used to measure redox reactions, such as the detection of glucose using glucose oxidase. In this case, the enzyme is separated from the electrode by a thin membrane that is only permeable to oxygen. Further, the enzyme is retained next to the electrode by means of a membrane, which is permeable only to low molecular weight molecules, including the analyte and the products of the enzyme action.
Conductimetric Biosensors
These biosensors detect changes in electrical conductivity produced by changes in concentration of ionic species. A typical example of such a biosensor is the urea biosensor based on immobilized urease. The reaction gives rise to a large change in ionic concentration, which is detected by the sensor.
An alternating field between the two electrodes allows the conductivity changes to be determined; this also minimizes the undesirable electrochemical process. The concentration of urea can be determined within the range 0.1-19mM; the assay tells a few seconds.
The method can be easily extended to reactions catalyzed by amidase, decarboxylases, esterase, phosphatases, and nucleases.
Optical Biosensors
These biosensors measure both catalytic and affinity reactions. They monitor fluorescence or absorbance changes caused by catalytic reaction products. Alternatively, they measure changes in the optical properties of the biosensor surface induced by dielectric molecules like proteins. A promising luminescence-based biosensor detects bacteria in food or clinical samples using the firefly enzyme luciferase.
Optical biosensor has been developed that detects parts per million of protein antigens using the specific antibody bound to the sensor. Similarly, complementary DNA/RNA can be attached to biosensor, which can now detect as low as few femtograms of complementary DNA as well as measure the rate of hybridization.
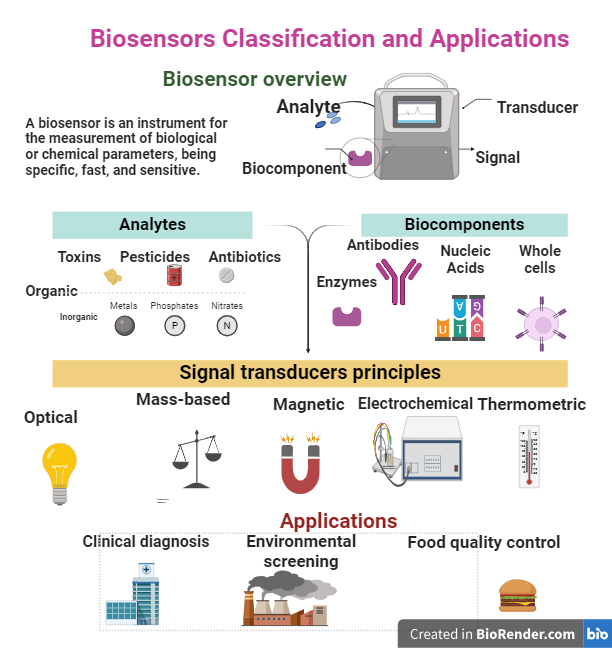
Fig: Biosensor, Types and its applications
Electrochemical Biosensor
The wearable biosensor is a digital device worn on the human body in various wearable systems such as smartwatches, smart shirts, and tattoos that allows for the measurement of blood glucose, blood pressure, heart rate, and other parameters.
For humans, these sensors may aid in the early detection of health events and the prevention of hospitalization. The likelihood of these sensors reducing hospital stays and readmissions will undoubtedly raise positive awareness in the near future.
Acoustic Wave Biosensors
These are also called piezoelectric biosensors. Certain crystals contain positive and negative charges, which separate, when they are subjected to stress. This generates an electric field, which is called piezoelectric effect. Such piezoelectric crystals can be used to assay the mass of an analyte that binds to the biological component immobile on the crystal surface.
For example, cocaine in the gas phase is detected by attaching cocaine antibodies to the surfaces of a piezoelectric crystal. This biosensor changes resonant frequency by about 50 Hz for one part per billion cocaine in the sample atmosphere. The biosensor can be reused after flushing it for a few seconds with clean air. A piezoelectric biosensor has been developed for the detection of enterobacteriaceae.
Whole cell Biosensors
Live or dead microbial cells can be used as the biological component of the biosensor in the place of the usual enzymes. The cell is cheaper, has a longer active lifetime and is less sensitive to inhibition, pH, and temperature variations than are isolated enzymes.
They are particularly useful where multi-step or coenzymes requiring reactions are necessary. But the biosensors based on whole cells usually have longer response and recovery times, a lower selectivity and are prone to biocatalytic leakage. Whole cells have been used to develop amperometric, potentiometric, thermometric devices.
Magnetic Biosensors
These sensors are used to monitor changes in magnetically induced effects or magnetic characteristics. These sensors use superparamagnetic crystals or particles to detect biological communications by sensing changes in magnetic properties such as coil inductance and resistance.
Comparisons among different types of Biosensors:
Featurers | Amperometric | Conductimetric | Potentiometric | Piezoelectric | Thermometric | Thermometric |
Cost | Low | Very low | Low | Low | High | Low |
Reliability | High | Medium | Medium | Low | High | Low |
Sensitivity | Medium | Medium | Medium | Low | High | Medium |
Complexity | High | Low | Medium | Low | Very high | Medium |
Speed of response | Medium | Medium | Medium | Fast | Slow | Medium |
General utility | Small | Small | Medium | Narrow | Wide | Small |
Current usage | High | Medium | High | Small | Medium | High |